Portland Limestone Cement: Improving Sustainability in Concrete Construction
As the nation, and the world, move forward in developing our built environment, achieving high standards in sustainable construction will literally and figuratively rest on concrete. Consider the fact that buildings are responsible for 39% of carbon emissions, 11% of which is attributed to the embodied carbon of materials and construction. On top of this already significant challenge to stabilizing global warming, the U.S. is embarking on a massive $1.2 trillion investment in infrastructure projects and anticipates adding 121 billion square feet of buildings by 2050. Considering all these climate-change contributors, the impacts on the planet’s vital ecosystems are tremendous.
Due to its significant lifespan, durability, strength and cost advantages, concrete is the most widely used material for building the foundations of our green-energy systems, climate-resilient infrastructure, and high-performance buildings. When it comes to embodied carbon, however, this essential building material has a large footprint primarily due to the inclusion of ordinary portland cement (OPC), the production of which is responsible for 8% of the world’s anthropogenic CO₂ emissions. Given concrete’s widespread use, specifying alternative sustainable cementitious materials to reduce the amount of traditional OPC used is a critical step in reducing the massive carbon footprint of the built environment.
Due to its significant lifespan, durability, strength and cost advantages, concrete is the most widely used material for building the foundations of our green-energy systems, climate-resilient infrastructure, and high-performance buildings. When it comes to embodied carbon, however, this essential building material has a large footprint primarily due to the inclusion of ordinary portland cement (OPC), the production of which is responsible for 8% of the world’s anthropogenic CO₂ emissions. Given concrete’s widespread use, specifying alternative sustainable cementitious materials to reduce the amount of traditional OPC used is a critical step in reducing the massive carbon footprint of the built environment.
Making Concrete More Sustainable
The easiest solution to reducing concrete’s carbon intensity is to replace Type I/II OPC in mixes with the more sustainable and similar-behaving Type IL Portland Limestone Cement (PLC). Holcim and many other U.S. manufacturers have now fully converted most of their plants and the markets they serve to PLC exclusively. Producers who have not yet made this necessary transition are expected to, driven by increasingly intensified stakeholder demands for building better with low-carbon building products.
Whereas the U.S. standard for portland cement (ASTM C150) allows up to 5% limestone in typical Type I/II cement, specification standards for blended cements (ASTM C595 and AASHTO M240) allow up to 15% of the cement to be replaced by finely ground limestone. Most suppliers are currently at a 10% replacement level and are expected to move closer to 15% replacement as they upgrade their plants. At this maximum level, Type IL PLC reduces the CO2 footprint of concrete by 10%. This is highly significant considering that more than 400 million cubic yards of concrete were produced in the U.S. during 2022. Additionally, PLCs can be used to further reduce concrete’s carbon intensity through similar use of supplementary cementitious materials (SCMs), such as fly ash and slag cement, as OPC.
Proven Record, Widely Accepted
PLC has a long and successful history of field performance in a variety of exposure conditions and applications. It has been used in Europe for more than 35 years and it has been increasingly used in Canada at limestone levels up to 15% since the adoption of the CSA A3000 standard in 2008. It was introduced into ASTM and AASHTO U.S. specifications in 2012.
With the recent acceptance of PLC by CalTrans, 47 state departments of transportation now allow the use of PLC for their projects. Type IL cement is also widely included in building codes—such as the International Building Code, ACI 318 (Building Code Requirements for Structural Concrete), and ACI 301 (Specification for Structural Concrete). It is recognized by the International Code Council, Federal Aviation Administration, Army Corps of Engineers, and American Institute of Architects Master Specs.
Material Characterization
Type IL cement performs similarly to Type I/II cement in concrete largely because most of the cementitious makeup is OPC (85-95%) while the remainder is limestone. Despite their performance similarities, it is important to recognize that the limestone is not completely inert. It contributes to the development of the concrete’s paste microstructure and has been found to synergistically react with some SCMs to enhance strength and durability properties, particularly when fineness and chemistry of the finished cement are optimized by the manufacturer.
The Blaine fineness of Type IL cement is generally higher than Type I/II cement because limestone is a softer material than clinker and it has a finer particle size. When interground with clinker, the limestone particles constitute most of the smaller particles in the blended cement, resulting in an increased level of particle packing and improved particle size distribution (PSD). This provides a more well-graded assortment of particle sizes and an enhanced paste density in concrete. It is for this reason that PLCs are better controlled by PSD directly, in order to optimize performance using modern control methodology.
The smaller the limestone particle sizes, the greater the total particle surface area for products to come into contact and react to increase the rate of calcium silicate hydrate crystal growth (the main binding phase in concrete). The fine limestone particles act as nucleation sites, increasing the hydration rate of the calcium silicates at early stages. The limestone can also react with aluminate to form or stabilize carboaluminates, providing additional space-filling hydrates. The extent of this reaction increases as the fineness of the limestone increases and when PLCs are combined with slag cement or fly ash, leading to synergistic potential.3
Performance Properties
Decades of extensive research, laboratory testing and field evaluations by cement manufacturers, ready-mix producers, project owners, academia and specifying agencies have shown that PLC can provide equivalent or better performance than OPC in concrete mixes, as exhibited in the references below. As with any product, it is recommended that performance be evaluated on a case-by-case basis.
In an independent research study on 12 different OPC and PLC mix designs (with and without SCMs),1 test results (Table 1) demonstrated minimal differences in the properties of concrete produced with OPC compared to PLC with Blaine fineness values of 462 m2/kg or 515 m2/kg. The concrete produced with PLC having the highest Blaine fineness (560 m2 kg) showed faster setting, reduced bleeding and higher strengths at all ages compared to the other concrete mixtures.
Table 1: Properties of Concrete Produced with OPC and PLC1
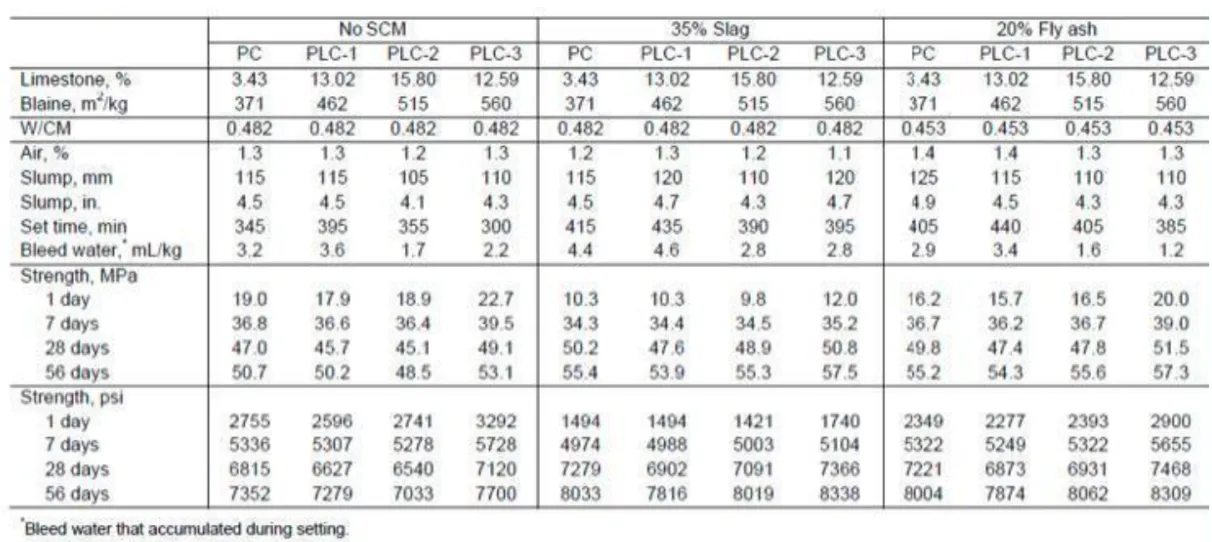
Below is a summary of the important fresh and hardened performance properties of concrete produced with Type IL PLC compared to concrete containing Type I/II OPC.
Workability. In general, the PSD of the Type IL cement is the main factor that influences the workability of a PLC concrete mix, like for like. PLC may slightly increase or decrease water demand and demand for chemical admixtures. A PLC concrete mix can be adjusted following the same workability approaches as those used for an OPC concrete mix (i.e., use of superplasticizers and other additives to achieve the desired results).2,3
Bleeding. The development of bleed water on the concrete surface is an important aspect of finishability and evaporation sensitivity. Bleeding is attributed to many factors, including water content, setting time, mix design proportions, particle size distribution and shape of any of the ingredients, and the depth of the concrete section, among others. As the surface area of the cement particles increases, water adhesion also increases and bleeding generally decreases. Based on its fineness, limestone use in cement can limit the migration of water and slightly decrease bleeding.3 While finishing best practices will not change, adjustments to mixture proportioning may require more attention.
Setting time. Increased fineness and levels of limestone generally have a neutral-to-slight accelerating effect on setting time, which can be addressed, if needed, through mixture proportioning. ASTM C150 outlines setting times of no less than 45 minutes and no greater than 6 hours, 15 minutes for Type I cement. ASTM C595 outlines setting times of no less than 45 minutes and no greater than 7 hours for Type IL cement.
Strength. Extensive testing by Holcim and other organizations has shown that PLC concrete can achieve similar strengths compared to OPC concrete when equivalent materials are used (Figure 1). Additionally, limestone levels of up to 15% can increase early-age strength due to improved particle packing, nucleation site effect, and production of calcium carboaluminate (Figure 2).1 Slightly increased long-term strengths have also been reported as the limestone’s Blaine fineness increases.3
Figure 1. Compressive strength comparison of Type I/II OPC vs. Type IL PLC produced at the Holcim Ste. Genevieve Cement Plant in Missouri.
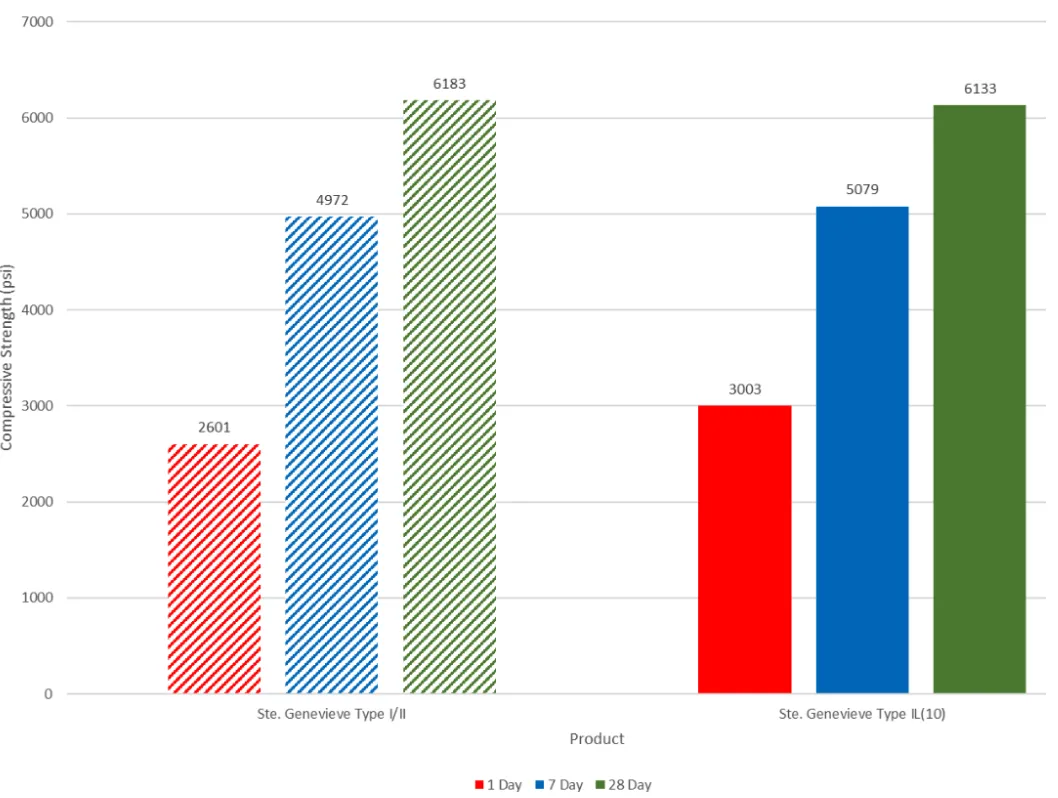
Figure 2. Early age strength gain of OPC and PLC concrete mixes with and without SCMs as reported in an independent research study by Thomas and Hooton.1
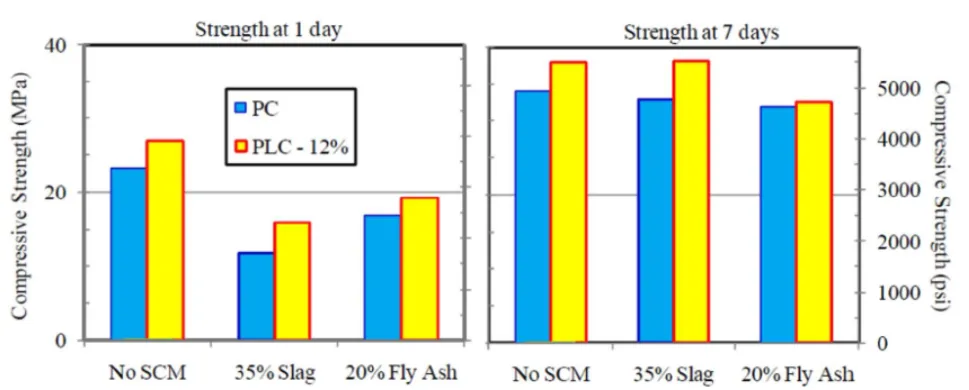
Permeability. Concrete produced with Type IL cement has been shown to provide similar or better resistance to the penetration of water, air, and accompanying aggressive chemical compounds—such as chlorides and sulfates—through enhanced pore structure in the cement paste (Figure 3).1-4 PLC concrete’s similar to lower permeability compared to OPC concrete is attributed to enhanced particle packing, paste density and chemical reactions of the limestone with aluminate to produce durable carboaluminate crystals.
Figure 3. Permeability performance of OPC and PLC concrete mixes with and without SCMs as reported by Thomas and Hooton.1
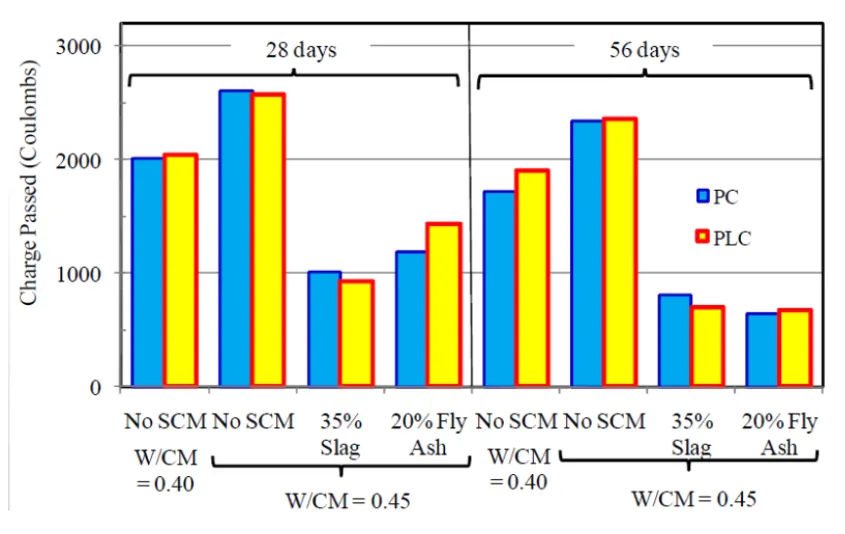
Shrinkage and creep. Drying shrinkage occurs primarily due to the capillary pressure that develops as fluid leaves the cement paste pores. Studies have shown that concrete produced with PLC—with or without SCMs—provides similar or slightly reduced shrinkage and shrinkage cracking than concrete made with OPC.3,5,6,11 Data collected on shrinkage from a study by the cement industry on Type I and Type IL cements are shown in Table 2. In addition, the creep characteristics of concrete produced with PLC are similar to concrete containing OPC.5
Table 2: Percent Shrinkage of Concrete Prisms (using ASTM C157) Produced with OPC and PLC*
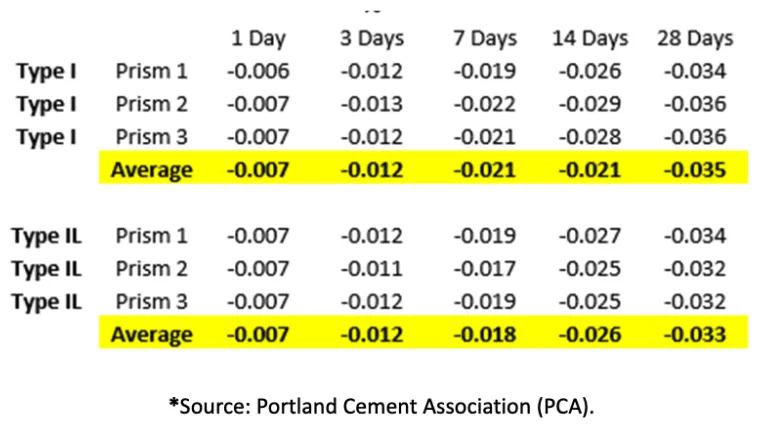
Alkali-silica reaction. Tests performed on mortar bars and concrete prisms containing alkali-silica reactive aggregates have demonstrated that properly proportioned concrete mixes produced with PLC resist ASR associated expansion similar to OPC concrete.2,3,7 Research studies have also shown that PLC concrete mixes provide an equal or higher level of resistance to ASR related expansion and cracking than OPC mixes in the presence of SCMs.2
Freeze-thaw durability and scaling. The accumulative effect of successive freeze-thaw cycles can eventually cause expansion and cracking, scaling, and crumbling of the concrete. Data collected on freeze-thaw resistance and deicer salt scaling by several studies on PLC concretes have shown no consistent difference between the behavior of equivalent OPC mixes.1-3
Heat of hydration. Thermal stresses develop as heat is generated during cement hydration. If the temperature differential between the concrete surface and interior is too high, cracking and loss of structural integrity can result. Studies have shown that heats of hydration are generally the same or decrease slightly when the PLC is used as a replacement for OPC. A slight increase in heat of hydration may be observed in some cases at early ages (generally up to 48 hours), but these differences are less significant at later ages.2,3
Contributing to a More Sustainable Future
The construction industry needs to act now in embracing innovative solutions that reduce the global warming potential (GWP) of building materials. One proven solution for reducing the embodied carbon of concrete by up to 10%—without sacrificing performance—is to seamlessly switch from OPC to PLC. Similar percentages of SCMs also can be used to further reduce OPC clinker in concrete mix designs.
Widely adopted by industry standards and building codes as a similar product to OPC, Type IL PLC is used throughout the U.S. as a direct one-to-one replacement for Type I/II OPC. As with any material changes to concrete, slight behavioral nuances of Type IL PLC will likely require adjustments to the mix proportion in some manner (water reducer, air entrainment agent, etc.) and should be monitored.
As the building sector progresses in its journey to decarbonize by midcentury, the market will increasingly move to more SCMs, blended cements and performance cements. Products are evolving and the availability of materials, such as fly ash, is continuously changing. It’s important that all industry stakeholders take the next steps in ensuring a smooth transition to the most sustainable solutions. These actions include evaluating the various material options available, requesting environmental product declarations (EPDs) from suppliers, and updating project specifications with current ACI guidelines on these sustainable solutions to help meet Architecture 2030 and SE 2050 climate-action commitments. Often, the best approach is to move from a prescriptive-based specification to a performance-based one. This will provide greater value to all industry stakeholders.
As an innovative leader in building materials, Holcim is committed to helping our customers specify and use optimal concrete mix designs incorporating our widely available OneCem® PLC product—along with our ECOPlanet™ portfolio of SCMs and blended cements—for achieving high performance and sustainability goals. In addition, we are constantly evaluating the viability of new materials and technologies to see how we can continue making our range of cementitious product offerings more sustainable. Contact your sales representative to learn how our technical experts can assist you with consultative advice, detailed test results, EPDs, quality control records and other support services.
At Holcim, we understand there may be concerns with the Type IL transition and we are prepared to support you in moving towards lower carbon cements.
Download the brochure to see a summary of some challenges that have been relayed and potential solutions
References
- Thomas, M.D. A.; Hooton, R.D., “The Durability of Concrete Produced with Portland-Limestone Cement: Canadian Studies”, Portland Cement Association, Skokie, IL, 2010, 28 pages.
- CALTRANS, “Impact on the Use of Portland-Limestone Cement on Concrete Performance as Plain or Reinforced Material,” 2021.
- Tennis, P.; Thomas, M.D.A.; Weiss, W.J., “State-of-the-Art Report on Use of Limestone in Cements at Levels of up to 15%,” SN3148, Portland Cement Association, Skokie, IL, 2011, 78 pages.
- Berke, N. S.; Inceefe, A. I.; Kramer, A.; Antommattei, O. R.; “Durability of Portland Limestone Cement Concrete,” Concrete International, Vol. 44, Issue 1, pp 34-39, 2022.
- Dhir et al., “Evaluation of Portland Limestone Cements for Use in Concrete Construction,” Materials and Structures. Vol 40, 2007, pp 459-473.
- Barcelo, L.; Thomas, M.D.A.; Cail, K.; Delagrave, A.; Blair, B., “Portland Limestone Cement Equivalent Strength Explained”, Concrete international, 2013, p 41.
- Laker, T.; Smartz, B., Evaluation of Portland limestone performance cements in 3137 Colorado and Utah transportation projects: 2007 to present, in Transportation Research 3138 Board, 2012.
- Thomas, M.; Cail, K.; Blair, B.; Delagrave, A.; Barcelof, L.; “Equivalent Performance with Half the Clinker Content Using PLC and SCM”, NRMCA Concrete Sustainability Conference, 2010.
- Hooton, R.D.; Nokken, M.; Thomas, M.D.A., “Portland-Limestone Cement: State-of-the-Art Report and Gap Analysis for CSA A 3000,” SN3053, Cement Association of Canada, Ottawa, ON, Canada, 2007, 59 pp.
- Barrett, T.J.; Sun, H.; Weiss, W.J., “Performance of More Sustainable Cements that Include Interground Limestone Additions of up to 15%,” Joint Transportation Research Program, Indiana Department of Transportation and Purdue University, West Lafayette, IN, 2013.
- Barrett, T.; Sun, H.; Villani, C.; Barcelo, L.; Weiss, J.; “Early-Age Shrinkage Behavior of Portland Limestone Cement,” Concrete international, Vol. 36, Issue 2, pp 51-57, 2014.